Introduction to Flare Gas Measurement
In oil and gas facilities, flaring is used as a safety mechanism to burn off excess hydrocarbons and relieve pressure during upset conditions. Monitoring the gas flow in flare stacks is crucial for several reasons. It enables operators to account for lost product and energy, close gaps in plant mass balance, and quantify greenhouse gas (GHG) emissions for environmental compliance (controlglobal.com). Many regulators now mandate accurate flare gas measurement – not only to track emissions but to avoid waste; failure to measure and report flared volumes can result in penalties. This focus on measurement aligns with industry decarbonization efforts: for instance, India’s largest producer ONGC has pledged to cut routine flaring and achieve zero methane emissions by 2030 as part of its climate goals (m.economictimes.com). Clearly, reliable flare monitoring has become an operational and environmental necessity.
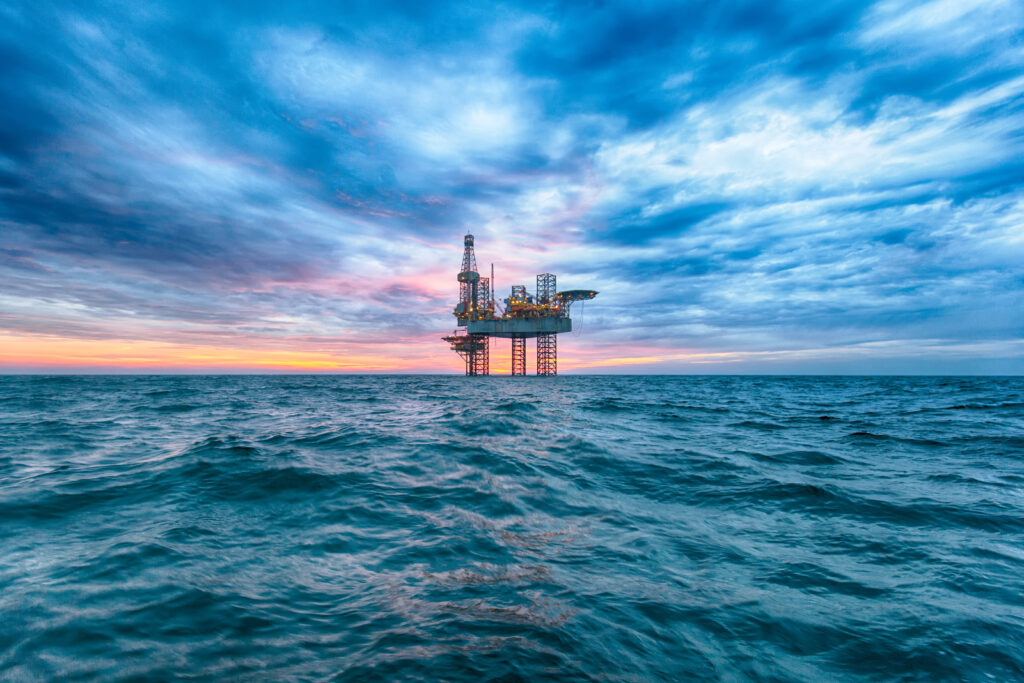
Flare gas measurement involves determining the flow rate (and often composition) of the gas being burned at the flare. Unlike normal process streams, flare flows are not steady or homogeneous – they can range from a small pilot flame gas to massive emergency releases, and the gas mix can change day by day. The measurement solution must therefore be highly adaptive and robust. In practice, various technologies exist (thermal mass flow meters, differential pressure devices, ultrasonic flowmeters, etc.), but each faces difficulties in the flare context. Ultrasonic flowmeters have emerged as a leading choice due to their ability to handle the extreme conditions of flare systems. Before exploring the ultrasonic approach and new innovations from Fluenta, it is important to understand what makes flare gas measurement so challenging.
Flare Gas Environments
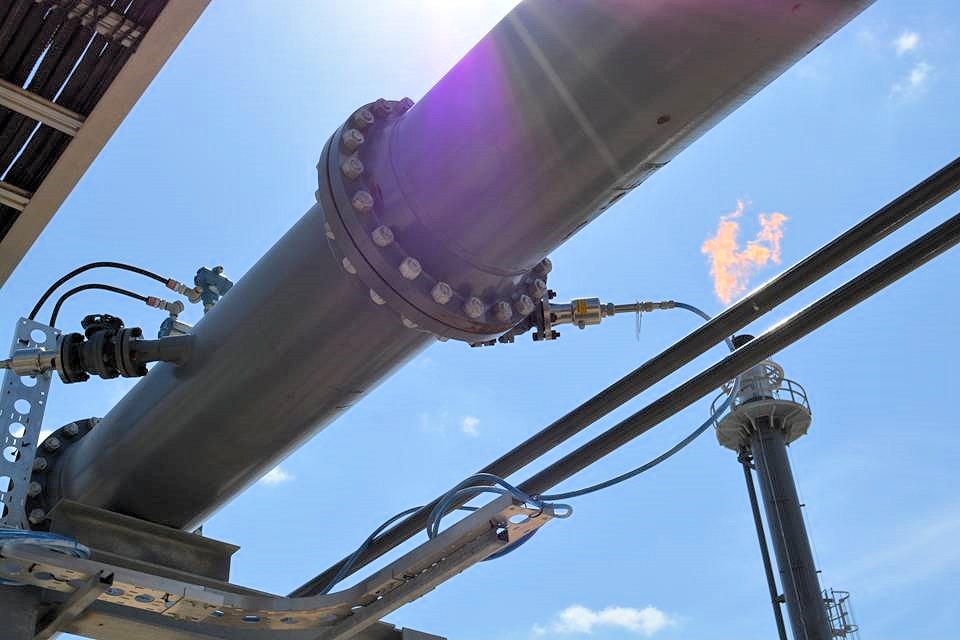
Flare gas systems present uniquely harsh and dynamic conditions that push flow measurement instruments to their limits. Key challenges include:
- Rapidly changing gas velocities: Flare gas flow rates can vary by orders of magnitude. In normal operation, flow may only be a trickle to keep the pilot flame lit, but during an emergency depressurization or blowdown, gas surges can exceed 120 m/s (meters per second). Such high velocities can carry over droplets of liquid and solid particles (e.g. sand, soot) that were not fully knocked out, creating a dirty flow. Instruments that protrude into the pipe can be bent or damaged by these high-speed particles. The measurement device must therefore handle both extremely low and extremely high flow rates – sometimes referred to as a wide turndown ratio – and withstand potential impacts or erosion from entrained debris.
- Variable gas composition (including CO₂ and hydrogen): Flare gas is rarely a single, consistent gas; it’s often a mixture of hydrocarbons and inert gases that fluctuates with process conditions. For example, a flare header might receive methane-rich gas one moment, then CO₂-heavy gas during a carbon-capture process upset, or even hydrogen-rich off-gas from a unit. Traditional meters calibrated for one gas can become inaccurate or inoperable when the composition shifts. High concentrations of carbon dioxide and hydrogen pose unique issues. CO₂ is a strong absorber of ultrasonic signals, dampening sensor readings and complicating flow measurement. Hydrogen, on the other hand, has an unusually high speed of sound (~1300 m/s) and tends to disperse widely, which makes capturing a clear signal challenging. These composition swings can lead to compliance issues if the meter can’t keep up. Overall, any flare flow measurement solution must either compensate for changing gas properties in real time or risk large errors when the gas mix deviates from the assumed calibration.
- High temperature and pressure fluctuations: Flare lines often operate near ambient pressure and temperature during purge or pilot flow, but they can see extreme conditions in certain scenarios. A sudden release of process gas may come at elevated pressure and temperature – for instance, flares connected to units like reformers or chemical reactors might handle gas well above 200 °C. In some industries (steel manufacturing off-gas, ammonia production, etc.), flared gas can routinely reach temperatures of 300 °C or more, far beyond the limits of standard instrumentation. Likewise, pressure in a flare header can spike during a major venting event. These temperature and pressure swings can cause physical stress and measurement drift in many flowmeter designs. The device must maintain accuracy despite thermal expansion, acoustic velocity changes, and other effects of a changing thermodynamic state. Any new flare metering technology is expected to withstand extreme heat and pressure without frequent recalibration or damage.
Given these challenges – wide velocity range, variable composition, and fluctuating conditions – it’s evident why flare metering is difficult. The ideal solution should handle all of these issues simultaneously: measuring tiny flows and giant bursts, coping with any gas mix, and surviving harsh environments. Ultrasonic flowmeters have become popular in this role because they fundamentally address many of these needs. Next, we discuss how ultrasonic flare meters work and why they are well suited to flare applications.
Ultrasonic Measurement Fundamentals
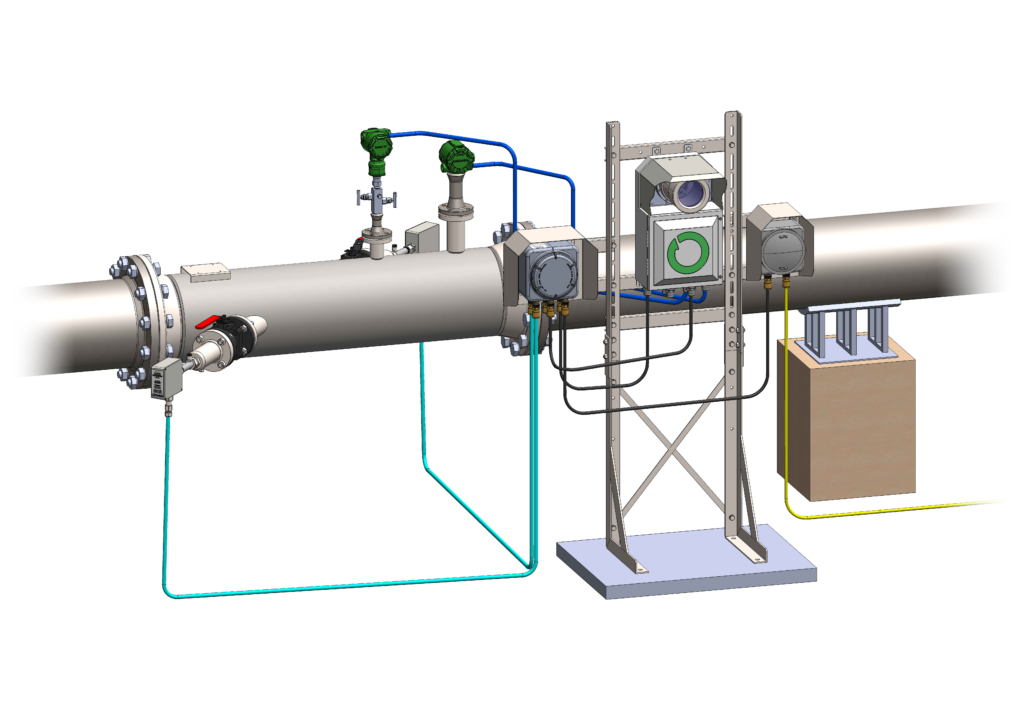
Ultrasonic flowmeters measure flow using high-frequency sound waves. In a typical flare gas ultrasonic flowmeter, a pair of transducers is mounted diametrically on the pipe (often at a 45° angle to the flow direction) . One transducer sends an ultrasonic pulse through the gas to the other side of the pipe. By alternately transmitting in the direction of flow and against the flow, the meter measures the difference in transit time between the two directions. This time difference is directly proportional to the gas velocity – much like a boat takes longer to row upstream than downstream(aiche.org) . Using the transit-time data along with inputs from pressure and temperature sensors, the flow computer can calculate both volumetric flow and mass flow of the flare gas. Modern ultrasonic systems update these calculations multiple times per second, providing a real-time flow rate. The method is purely electronic and acoustic, with no moving parts in the flow. Because of their precision, ultrasonic flowmeters are not only used for flare monitoring but have even been accepted in fiscal metering (custody transfer) applications, attesting to their accuracy and repeatability.
Key advantages of ultrasonic flare flowmeters include:
- Wide turndown and high accuracy: Ultrasonic meters can measure a very broad range of flow rates with one device. They are sensitive enough to detect very low flow velocities (on the order of a few centimeters per second) yet robust enough to track velocities over 100 m/s. For example, Fluenta’s standard flare ultrasonic meter offers a turndown ratio of 4000:1 (covering flows from 0.03 m/s up to 120 m/s) without sacrificing accuracy. This wide rangeability means a single ultrasonic meter can catch small leaks or purge flows and also handle massive emergency flaring events. Ultrasonic flow measurements are highly reproducible and accurate; performance on the order of ±1–2% of reading is achievable, which is why they are deemed suitable even for custody transfer of gas. High accuracy across a wide operating envelope gives operators confidence in the data for both compliance reporting and operational decision-making.
- No intrusions or moving parts (reliability): Unlike pitot tubes or turbine meters, ultrasonic transducers do not obstruct the pipe’s cross-section – they can be mounted flush with the inner wall, or even clamped externally on some designs. This non-intrusive approach means negligible pressure drop and no risk of creating a trap for debris. With no rotating gears or vanes, there’s nothing to wear out or seize; maintenance requirements are minimal. The transducers themselves are typically the only wetted components and are built to withstand exposure. Even if a layer of condensate or soot deposits on the sensor, it usually only attenuates the sound signal slightly rather than stopping measurement. In contrast, an intrusive probe can become coated (fouled) and then give erroneous readings until cleaned. Ultrasonic meters’ tolerance to dirt and lack of moving parts translate to high reliability and long service life in flare applications. The design also avoids placing fragile components in the direct blast of high-velocity gas, so damage from sudden surges is far less likely.
- Real-time adaptation to gas composition: A unique feature of ultrasonic technology is that it inherently measures the speed of sound (SoS) in the gas (as part of the transit time calculation). Because the speed of sound through a gas depends on the gas’s molecular weight and composition, the ultrasonic meter can use the measured sound velocity to infer the average molecular weight of the flare gas continuously (controlglobal.com). In practice, this means the meter can automatically compensate for changing gas composition on the fly. For instance, if the flare gas shifts from light methane to heavier propane-rich gas, the ultrasonic meter “sees” the change via a change in sound speed and adjusts the density calculation accordingly. The result is a more stable and accurate flow reading without needing manual recalibration for each new gas mixture. This capability is extremely valuable in flare systems where composition may be unpredictable – it allows ultrasonic flowmeters to maintain accuracy where other technologies would mis-measure or require frequent reprogramming. Indeed, industry studies (including API guidelines) note that while thermal meters can err by 5–15% or more under changing flare gas compositions, ultrasonic volumetric readings remain essentially unaffected by those composition changes (fluenta.com).
- Diagnostics and digital integration: Ultrasonic flowmeters often come with advanced self-diagnostic features. They can monitor parameters like signal strength, signal-to-noise ratio, and transit time consistency to assess if the meter is performing correctly (controlglobal.com). This provides an online way to verify meter health and data quality, without pulling the meter out of service. For example, if fouling begins to interfere with the signal, the meter’s automatic gain control will increase – a parameter that maintenance personnel can monitor remotely. Such diagnostics help schedule maintenance proactively and ensure compliance (since any degradation can be detected and rectified). Additionally, ultrasonic flare meters typically output digital data (via protocols like Modbus, HART, etc.), making it straightforward to integrate into plant control systems and emission monitoring software. This digital connectivity complements modern Continuous Emissions Monitoring needs by feeding real-time flow data into broader environmental data systems.
In summary, an ultrasonic flare gas meter offers a combination of accuracy, rangeability, and robustness that is well-suited to the task of flare monitoring. These benefits have led to ultrasonic technology becoming the preferred solution in many flare applications, especially as environmental regulations grow stricter. However, it is useful to compare this approach with other available technologies to understand the trade-offs.
Comparison with Other Technologies
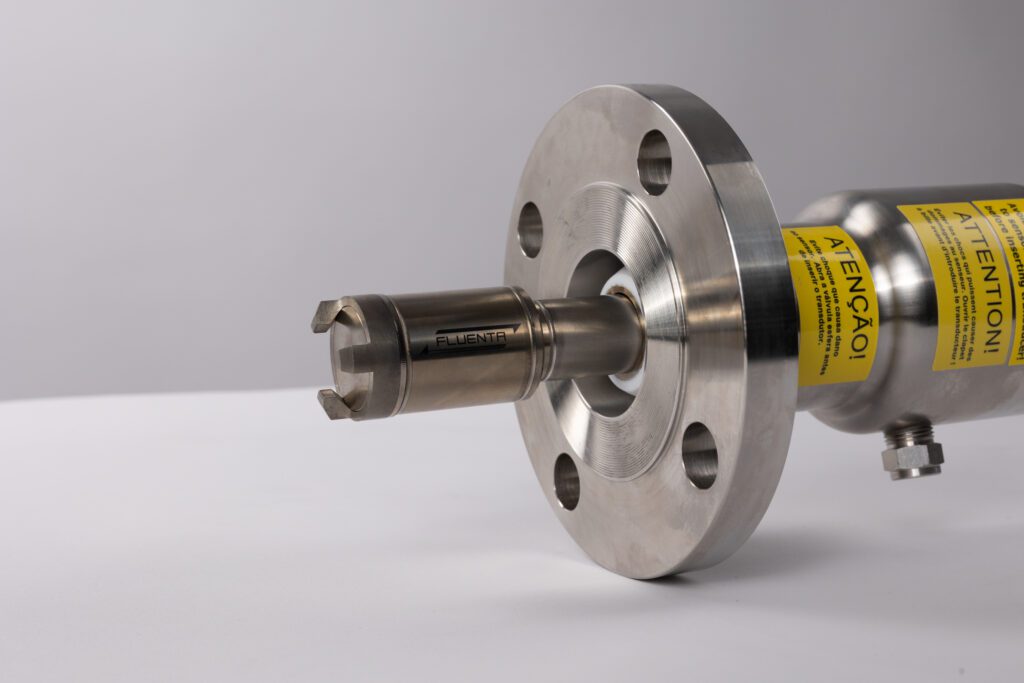
Historically, several flow measurement technologies have been applied to flare gas, each with pros and cons. The demanding nature of flare service tends to expose the limitations of many conventional meters. Below is a comparison of ultrasonic meters with other common technologies for flare gas flow measurement:
- Thermal mass flow meters: Thermal dispersion flowmeters measure mass flow by heating a sensor and gauging how much heat is carried away by the gas flow. They are relatively simple and work well if the gas composition remains constant. However, in flare service the gas makeup is rarely stable, which is a major drawback. Thermal meters must be factory-calibrated for a specific gas mixture; when the composition changes, their readings become inaccurate unless corrected (aiche.org). Modern thermal meters can use an attached gas analyzer to periodically adjust for composition, but this introduces latency – a flaring event might be over by the time the analyzer updates the meter (aiche.org). Fast transients or unusual gases (like hydrogen) can cause huge errors. For example, an API study showed that switching a thermal meter from a methane-based calibration to a high-hydrogen gas could induce errors exceeding 100–300% (fluenta.com). In contrast, an ultrasonic meter’s reading of volumetric flow was essentially unchanged by the same composition shift (fluenta.com). Thermal meters also typically have intrusive probes that sit in the flow. These can be damaged by high velocity particles or get coated by condensates, which skews the readings(fluenta.com). Removing a thermal meter for cleaning usually requires a process shutdown(aiche.org). In short, while thermal mass flowmeters are useful in some gas flow applications, flare conditions (with widely varying gas mixtures and dirty, high-velocity flow) push them beyond what they handle well without frequent recalibration or maintenance.
- Differential pressure (DP) meters: This category includes devices like orifice plates, Venturi tubes, and averaging pitot tubes that create a pressure drop correlated to flow rate. DP meters are a long-established technology with well-defined standards and are even used in custody transfer in other applications (aiche.org). They function reliably for steady flows of known fluid properties. In flare service, though, DP meters face multiple limitations. First, they have a limited turndown ratio – the relationship between flow and differential pressure is non-linear, and at very low flow the DP signal is so small that accuracy suffers. A DP meter sized for a huge blowdown event will not read a tiny purge flow accurately. Second, DP devices inherently consume pressure; an orifice plate for instance creates a permanent pressure drop, which in a flare line is undesirable because it can inhibit flow during an emergency. Some DP elements might use up 0.5 psi or more of line pressure to measure properly (controlglobal.com), which is significant if the flare system operates near atmospheric pressure. Third, these meters often have intrusive components (plates, impulse lines, etc.) that are prone to plugging or fouling over time (aiche.org). In a flare carrying oily residue or water vapor, an orifice can get dirty, altering its calibration. Pitot tubes can clog with particles (azom.com). Thus, DP meters would require frequent inspection and recalibration in flare service (controlglobal.com). Finally, like thermal devices, DP meters are affected by gas density – a DP flowmeter calibrated for a certain gas composition will give errors if the actual gas density changes significantly (e.g. high CO₂ content) unless the readings are corrected. Overall, while DP flowmeters are well understood, their poor turndown, pressure drop, and fouling susceptibility make them less ideal for flaring applications compared to ultrasonic technology.
- Mechanical flowmeters (turbine, vortex, etc.): Older mechanical metering technologies are generally not suited to flare gas for a variety of reasons. Turbine flow meters, which use a spinning rotor in the flow, cannot handle the huge range of flow rates in flaring. They tend to stall at low flow (the rotor stops turning below a threshold) and can overspeed or even break at extreme high flows. Turbines also have moving parts that wear and require regular maintenance or calibration. In fact, industry guidelines explicitly state that turbine flow meters are not recommended for flare gas measurement (assets.publishing.service.gov.uk). Any meter with moving parts in a potentially dirty, intermittent flow is a liability – bearings can corrode or gum up, and a slug of liquid in the flare could wreck the internals of a turbine meter. Vortex shedding flowmeters (which measure vortices shed off a bluff body in the flow) have no moving parts, but they suffer other issues in flares. Vortex meters need a certain minimum flow velocity to generate stable vortices, so like DP meters they struggle with low-flow sensitivity and turndown. They are also vulnerable to fouling – if the bluff body gets coated with deposits, the vortex formation can be disrupted, leading to inaccuracies (controlglobal.com). Vortex meters also typically have an upper limit on flow rate frequency; beyond a point, they can’t count vortices accurately, so a single meter might not cover a full flare range. Additionally, neither turbines nor vortex meters can inherently correct for changing gas composition (they measure volume and would need external density compensation to report mass or standard volume). Given these drawbacks, mechanical flowmeters are seldom used for flare gas monitoring today. The consensus is that ultrasonic flowmeters provide far superior performance under the variable and extreme conditions of flaring, with far less maintenance overhead (aiche.org).
(It’s worth noting there are other niche technologies like optical flowmeters and Coriolis meters. Optical flowmeters use lasers or imaging to infer flow and can be non-intrusive, but they tend to foul easily in flare service and need clean optics (aiche.org). Coriolis meters measure mass flow directly and are very accurate for stable flows, but they are generally impractical for large flare lines due to high cost and sensitivity limitations – in fact, guidelines consider Coriolis unsuited to flare gas measurement in most cases (assets.publishing.service.gov.uk). As a result, ultrasonic systems have effectively become the industry standard for critical flare gas measurements.)
Fluenta’s 2025 Product Innovations
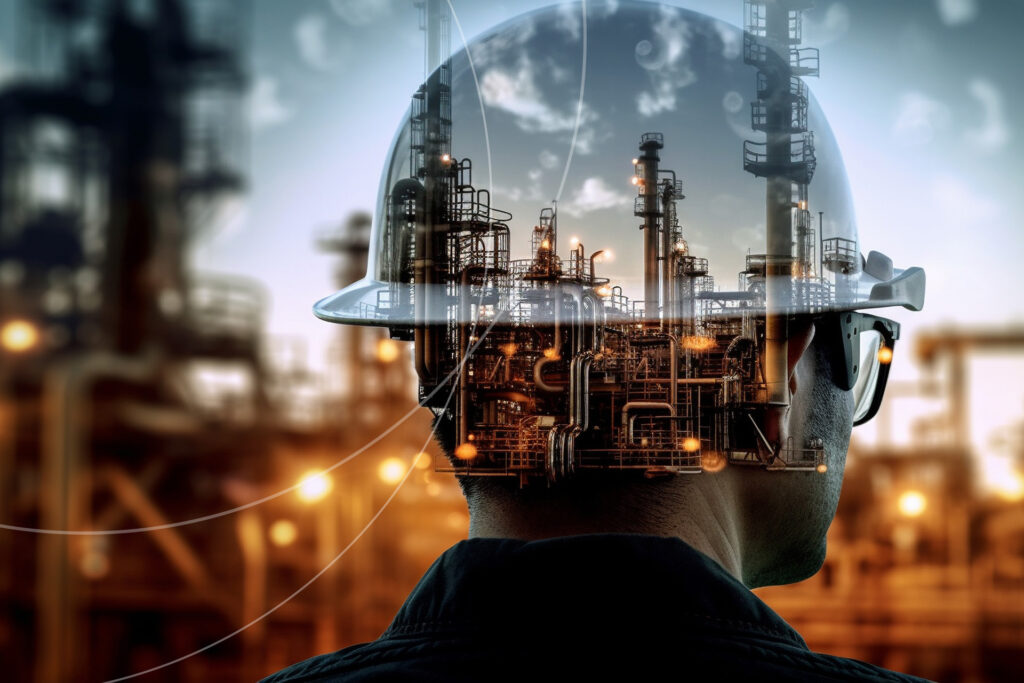
With decades of experience in ultrasonic flare monitoring, Fluenta has been at the forefront of addressing flare measurement challenges. In 2025, Fluenta introduced a new suite of product innovations designed to push the boundaries of what ultrasonic flare gas meters can handle. These developments specifically target the extreme scenarios of flare measurement: very high temperatures, highly CO₂-rich or hydrogen-rich gas mixtures, and the need for integrated emissions data handling. Below we highlight Fluenta’s latest solutions and how they tackle the problems outlined earlier:
- Ultrasonic sensors for very high temperatures: Fluenta’s newest FlarePhase high-temperature ultrasonic sensors are engineered for process conditions up to 350 °C and beyond (fluenta.com). This breakthrough directly addresses flaring in industries like petrochemical refining, steel manufacturing, or emergency relief events where gas temperatures soar well above the limits of standard ultrasonic transducers. The innovation lies in advanced materials and design: the sensor tips are made of titanium and specialized ceramics that withstand extreme heat, and the internal electronics are fortified for high-temperature operation. Moreover, the system actively monitors the sensor temperature and auto-calibrates the ultrasonic signal to maintain strength and accuracy. In effect, Fluenta has expanded the operating window of ultrasonic meters into ranges previously impossible – measuring reliably at 350 °C (as well as in cryogenic lows around -40 °C with the same device). This ultra-wide temperature capability is one of the widest operating ranges on the market. It ensures that even in flaring scenarios with intense heat (such as ammonia synthesis purge gas or flare stacks in hot climates), the ultrasonic meter will continue to perform. By overcoming resonant frequency drift at high temperatures, the FlarePhase high-temp sensors provide accurate readings without the need for cooling or special housing, simplifying installation in hot flare lines.
- Accurate measurement in CO₂-rich gas: Another 2025 innovation from Fluenta is a solution for flares containing very high levels of CO₂. Traditional ultrasonic meters had difficulty beyond ~30% CO₂ due to signal attenuation – the CO₂ molecules absorb sound energy, weakening the ultrasonic pulse. Fluenta’s response is the FlarePhase CO₂ technology, a combination of custom transducers and signal processing optimized for CO₂’s acoustic properties. This technology has been rigorously tested by an independent institute (IPT) across the full range of 0% to 100% CO₂, and it demonstrated successful flow measurements even at 99+% CO₂ concentration. The results showed high accuracy and repeatability at various flow rates and pipe sizes, meeting and even exceeding industry standards for flare metering (fluenta.com). In practical terms, this means operators can now deploy ultrasonic meters on flares in Carbon Capture, Utilization and Storage (CCUS) facilities or CO₂-heavy Enhanced Oil Recovery operations with confidence. Previously, such flares were a blind spot for ultrasonics, often forcing use of less accurate methods. Fluenta’s CO₂-optimized meter maintains a strong signal in CO₂-rich gas and counters the “ultrasonic signal absorption” challenge through a proprietary transducer design (branded FlarePhase). This is enabling reliable, real-time measurement in CO₂-rich flare gas where non-compliance or data gaps were a major concern before (fluenta.com). The technology ensures that even as CO₂ content fluctuates or spikes to 100%, flow data remains accurate – a critical capability as CO₂ monitoring becomes a regulatory requirement in many regions.
- Toward 100% hydrogen flaring measurement: Anticipating the industry’s shift towards hydrogen, Fluenta is actively developing solutions for flares handling high hydrogen content, up to pure H₂. Hydrogen presents a notoriously tough scenario for ultrasonic flow measurement. Its extremely high speed of sound (~1280 m/s) means the transit-time difference in a small ultrasonic path is minuscule, demanding ultrafast processing and high signal resolution (fluenta.com). Additionally, hydrogen’s acoustic behavior – low density, wide dispersion, and tendency for sound to reflect in piping – makes it hard to distinguish the direct ultrasound signal from noise. Fluenta’s R&D has focused on new electronics and digital signal processing (DSP) techniques to overcome these challenges. In 2024, Fluenta was selected as a partner in the UK government’s FutureGrid project, which is aimed at proving technologies for a future hydrogen gas network. Through this initiative, Fluenta is working to deliver a robust ultrasonic flare meter capable of accurately measuring 100% hydrogen flow within the next 18 months. This involves specialized transducers materials and processing algorithms to handle the rapid signal and unique acoustic profile of H₂. The goal is that by 2026, Fluenta will have a field-proven hydrogen flare measurement system, positioning it as one of the first to offer a solution for hydrogen flaring (which is relevant for hydrogen production facilities, refineries co-firing hydrogen, or any future scenario where hydrogen replaces natural gas streams). By tackling the “high-velocity hydrogen measurement predicament” head-on, Fluenta is ensuring that its ultrasonic technology remains at the cutting edge of the energy transition. Operators dealing with hydrogen can expect the same level of accuracy and reliability for flare monitoring as currently achieved with natural gas, thereby extending emissions monitoring and safety to hydrogen operations.
- Next-generation continuous emissions monitoring (CEM): Beyond the hardware improvements, Fluenta’s 2025 innovations include a new software and systems solution called FlareSens for emissions monitoring. FlareSens is a continuous emissions monitoring system (CEMS) that works in tandem with the ultrasonic flare meter to automatically collect, calculate, and report flare-related emissions data. In today’s regulatory climate, companies must keep detailed records of how much CO₂ and methane they emit via flaring, often under frameworks like the EPA’s GHGRP, the EU Methane Regulation, or voluntary programs like OGMP 2.0. FlareSens greatly simplifies this task. It combines the flow rate data from the ultrasonic meter with gas composition data (from gas analyzers or configured gas streams) to compute key metrics like total CO₂ emissions, methane emissions, and the net heating value of the flare gas in real time. All of this information is continuously logged and can be accessed through a user interface or exported to reporting software. Essentially, FlareSens provides a granular live view of emissions and automates the data capture needed for compliance reports. Operators no longer have to manually integrate flow totals with lab analyses at the end of the month – the system outputs ready-to-use emission figures. This not only reduces the reporting workload but also helps identify opportunities to reduce emissions. For example, FlareSens can reveal a persistent small flare flow (indicating a valve leak) or calculate combustion efficiency (so operators know if methane is slipping through unburnt). The platform is designed to meet emerging regulatory requirements and financial pressures; for instance, with methane emissions now being taxed in some jurisdictions (the U.S. Inflation Reduction Act imposes a fee rising to $1500/ton by 2026), having accurate, real-time methane data is crucial to avoid unexpected costs. By providing real-time insights and ensuring the data aligns with regulatory standards, Fluenta’s FlareSens acts as an enabling tool for operators to proactively manage flaring. It empowers users to make decisions (like adjusting operations to reduce flaring or justifying investment in flare gas recovery) based on reliable data. In short, the new CEM solution turns the flare meter into a comprehensive emissions management system – helping companies not only comply with reporting rules but actually drive emissions reductions through better visibility. As regulations tighten and public scrutiny of flaring increases, such integrated solutions offer a forward-looking way to stay ahead of both compliance and corporate sustainability goals.
Together, these innovations reinforce Fluenta’s position as a leader in ultrasonic flare measurement. By addressing high-temperature durability, CO₂ and hydrogen handling, and advanced emissions data integration, Fluenta is ensuring that oil and gas operators have the tools needed to measure flare gas accurately under any condition. For technical professionals in the industry, where there is a strong push for methane reduction and stricter environmental governance, these technologies provide a path to more reliable operations and better emissions control.
Conclusion
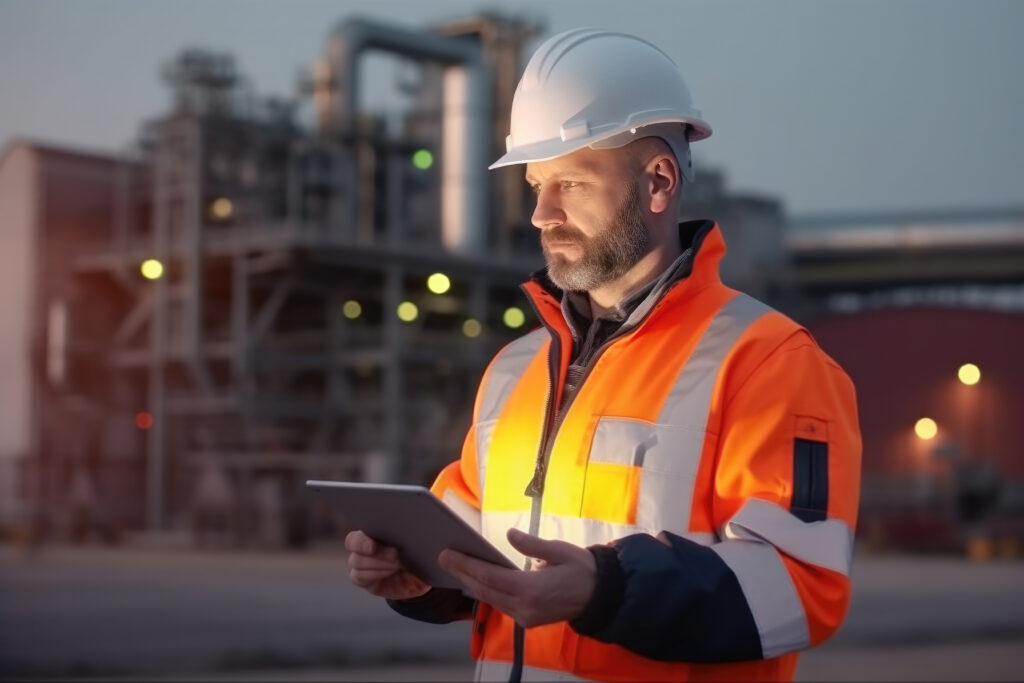
Flare gas measurement is a critical component of both operational efficiency and environmental responsibility in oil and gas operations. The unique challenges of flare systems (fast-changing flow rates, variable compositions, extreme conditions) mean that choosing the right measurement technology is vital. Traditional flowmeters struggle to cope with the wide dynamic range and unpredictability of flaring, often resulting in inaccurate data or high maintenance burdens. In contrast, ultrasonic flare flowmeters have proven to be particularly well-suited for this task, thanks to their high turndown, non-intrusive design, and ability to handle changing gas properties (aiche.org). They enable operators to accurately track flared gas volumes and thereby quantify emissions, satisfy regulatory requirements, and identify opportunities to reduce flaring. This accurate accounting is not only important for compliance (ensuring alignment with pollution control norms and climate pledges) but also for performance improvement – for example, by detecting unseen losses or improving flare combustion efficiency.
The latest developments from Fluenta in 2025 demonstrate how ultrasonic technology continues to evolve to meet new industry demands. Enhanced hardware now allows measurement in environments once considered too extreme (high temperature, pure CO₂ or H₂ streams), and intelligent software integration is turning data into actionable insight in real time. These advancements position Fluenta as a forward-thinking partner for oil and gas companies aiming to modernize their flare systems. With regulatory pressures mounting and corporate ESG commitments (such as methane reduction targets) on the rise, having a robust flare measurement and emissions monitoring setup is increasingly a best practice. Technologies like Fluenta’s ultrasonic flare meters and FlareSens CEM system give operators the confidence that every cubic meter of gas sent to the flare is properly measured and accounted for, even under the toughest conditions.
Wherever operators are balancing growth with sustainability, adopting such cutting-edge flare metering solutions can be a game-changer. It ensures transparency of flaring operations and helps align with global environmental standards and initiatives. By investing in accurate flare gas measurement today, companies not only avoid potential fines and meet reporting mandates, but they also gain the data needed to drive down flaring volumes tomorrow. Ultimately, precise flare gas measurement – as enabled by advanced ultrasonic technology – is a key enabler for safer, more efficient, and cleaner oil and gas production. It transforms the flare from a “black box” of unknown emissions into a monitored source of valuable information, guiding the industry toward its goal of reduced emissions and improved operational excellence. Ultrasonic flare gas measurement, backed by innovations from firms like Fluenta, stands ready to support this transition, offering reliability and insight when they are needed most.